
Complexity - Simplified (kind of!)
Both philosophy and science divide the world into easier-to-study-sized “chunks.” Philosophy is divided into metaphysics, ethics, epistemology, and politics to name a few of its major subdisciplines. Science, which before 1833 c.e., used to be called “natural philosophy,” is similarly divided into physics, chemistry, biology, psychology, and the like. Those disciplines are then divided, and often divided several more times. That’s fine of course if you want to become the expert in say, the physiology of sperm whales, or the business ethics of American non-profit organizations. At some point, however, we need to take a step back from this reductionist approach of understanding ever smaller parts of the world and put the pieces back together again.
A few disciplines are striving to understand the world by a more “holistic” or transdisciplinary approach, and complexity science is one of them. The reason for this approach is pragmatic as well as ideological – complex systems occur in multiple disciplines, including meteorology, biology, sociology, ecology, and technology to name a few. Philosophy also makes queries about the world in a fundamentally complex world as well. Ethicists for example, wants to know what is the right action for a person who is situated in a complex society existing on a complex planet (and the person is complex as well!). Indeed, the universe at large is fundamentally a complex system. Hence, the very things being studied cross multiple disciplines. Also, even if a scholar were to choose to study only the complexity of ant colonies for example, they would need to understand various disciplines just for that task, including statistics, physiology, entomology, and ecology for starters.
Complexity science strives to not only understand how complex systems become extant, but also their unique properties, how they function, sustain themselves, and come to manifest their unique phenomena. For example, why and how do 100,000 army ants work together to construct living bridges with their own bodies, overtake and consume much larger animals, and build living nests each night to protect the queen and her brood, whereas “only” 100 army ants will just wander around aimlessly until they die? What factors make national and international economies suddenly surge or collapse, the internet to occasionally crash, large wars to break out, ecosystems and humans societies to thrive or collapse, and so on? Before we examine some of the manifestations of complex systems, a.k.a. complexities, we need to try to define or describe what we mean by “complex.” (1)
What is “Complex?”
Complexity science began to be formally studied at the Santa Fe Institute in 1984. Despite intense and sophisticated research, authorities in this interdisciplinary field do not agree fully on a concise definition for “complexity.” However, they do generally agree that the following characteristics are necessary, even if not sufficient:
-
A complex system consists of multiple interactive components, often referred to as “agents.” These agents might be the varied white cells of an immune system, the neurons (also varied) of a brain, or traders participating in the stock market.
-
The agents exchange and process information amongst themselves without overall central control. A classic example of this process is a flock of birds or a school of fish that move in shifting formations without a central leader. Instead, each bird or fish is following rules regarding proximities to their neighbor. The lack of central control extends to other complex systems including brains and societies. Even though “you” might think that you are in control of your brain, it is in fact carrying out a myriad of functions like respiration, digestion, circulation, balance, sensory processing, etc., without your awareness. Similarly, even the most totalitarian government cannot manage every aspect of the members of its society.
-
Complex systems are dynamic, and, therefore, require free energy flow. “Dynamic” is a technical word for “changing.” Static systems like a parked car do not do anything “interesting” except decay with time due to entropy – the tendency for all systems to become disordered unless countered by ordering processes as occurs in a living organism. Dynamic systems, however, interacts both internally and externally with their environment, which requires work energy to accomplish. Because complex systems can only exist and function within a certain range of order, they also require energy to maintain that range of critical relationships amongst its constituents.
These are at least a partial list of the bare necessary elements of a complex system but, again, they are not sufficient because some entities that are not complex would also have these characteristics. For example, an expanding cloud of hot gas, has multiple components (atoms, molecules, and radiation) that interact with each other, even if only via collisions, and is expanding due to free energy flows. Complex systems, however, also typically have at least some of the following characteristics: (2).
-
They exhibit patterns of behavior that would not be predicted from the behaviors or characteristics of its more fundamental components. The phenomenon is usually referred to as “emergence.” For example, no matter how much you studied a neuron, even a “super-physicist-biologist-neuroscientist” would not predict that a collection of them put together in just the right way could eventually manifest self-awareness, write songs, fall in love, and solve math problems. Emergent phenomena can be abstractly represented as A+B+C→X, where some relationship that occurs between its components, represented as “A,”“B,” and “C” yields an emergent product “X.” The interacting components of a non-complex system usually results in a simple summation or conjunction that could be represented as A+B+C→ABC, where “ABC” is a new product, but with no unexpected properties.
-
They self-organize and self-regulate their structure and processes.
-
They exhibit “non-linear” behavior which makes them hard to predict, i.e., for any given inputs, the resulting outputs do not fall on a straight line, and the result can often only be predicted statistically, not with deterministic certainty.
-
Their structure and processes are neither too ordered, as with a quartz crystal, nor too random, as with a room of air molecules. Instead, they exist somewhere in between these two extremes.
-
Additionally, most authorities in complexity science include “adaptation,” as a requisite criterium or will divide complex systems into “non-adaptive” complex systems (e.g., hurricanes, the sun), and “adaptive” complex systems (e.g., living organisms, human society). In this context, adaptation means that something can alter itself or its successor(s) so that it improves its function, or so it is more likely to persist despite changes in its environment. Adaptation also serves as a “bright line” for complexity because only life and systems derived from it (e.g., ecosystems, the immune system, economies, the internet) can attain this quality, whereas non-living entities and their derived systems persist or do not persist according to the more fundamental rules of physics. Stars might last many billions of years, have countless interacting parts, and have emergent properties like nuclear fusion and the creation of new chemical elements, but it cannot alter its structure or processes of itself or its successors to better survive a change in its surroundings.
A pithy summation of one of the results of adaption was written by Erwin Schrödinger (1887-1961), the famed quantum physicist of “Shrödinger’s cat” fame, in his insightful 1944 book entitled “What is Life.” He famously stated:
"What is the characteristic feature of life? When is a piece of matter said to be alive? When it goes on ‘doing something’, moving, exchanging material with its environment, and so forth, and that for a much longer period than we would expect an inanimate piece of matter to ‘keep going’ under similar circumstances." (3)
Indeed, single cell living organisms, and even minimally differentiated multicellular organisms like sponges and the “immortal jellyfish” (Turritopsis dohrnii) are essentially immortal (4). For example, bacteria divide by fission so that while the vast majority of its successors do die, at least some typically persist to go on dividing. The evidence suggests that all bacteria and even higher life forms are descendant from “Last Universal Common Ancestor” (L.U.C.A.) from at least 3.6 billion years ago - even if L.U.C.A. has also subsequently altered itself in variety and degree of complexity a million-fold in the ensuing epochs.
This list of characteristics of complexity is far from exhaustive but captures most of its key elements. For the purposes of this website that is initially concerned with ethics, as well as with most discussions in the complexity literature and community, we will restrict complexity to those that are adaptive, and, hence, are living systems and systems derived from them.
Figure 2. An abstract representation a nascent complex system with 3 interacting components (e.g., quarks that interact to form a proton or neutron), each represented by a different colored square, their interactions by arrows, and with the circle encompassing the system itself.
Complex systems – Neither Too Ordered, nor Too Disordered and the Concept of “Relational Space”
The fourth characteristic of complex systems listed above warrants a bit more explication for our purposes: complex systems are neither too ordered like a quartz crystal, nor too random like the air moving in a room. Instead, they have the right amount of “chaos” to be interesting. “Chaos” in this context does not mean extreme randomness but refers to a system whose outcome is incredibly sensitive to “initial conditions.” Edward Lorenz (1917-2008), a meteorologist and mathematician is credited with accidentally discovering this key phenomenon of chaotic systems when he set up a computer to help with weather forecasting and discovered that even with a miniscule change in initial input parameters, large changes occurred in subsequent weather predictions – the so called “butterfly effect.” (5). Without going further into other often fascinating features of “chaos,” the primary point is that complex systems have a dynamism such that absolute deterministic outcomes are even in principle impossible to precisely predict, but still will usually fall within a certain range of values.
Because complex systems exist in a large, but finite number of possible states of relationships amongst its constituents, rather than just “essentially” one state (like a crystal) or a near infinite number of states (like a container of air), it is helpful for our purposes to depict complex systems as having this range of relationships which I will call a “relational space.” This conceptual space is similar to that of “phase space” used in the physics discipline of thermodynamics. “Relational spaces” differs from thermodynamics’ phase spaces because it includes the macroscopic as well as microscopic relationships of a system – like that of an arm to a shoulder or the hip to the leg. Figure 3 abstractly depicts the relational spaces of systems at different levels of “well-being.” If the relationships of a system fail (e.g., a person has an arm amputated, one farmer steals from his neighbor, a tornado destroys a room), then the complexity whether it is a person, an agrarian village, or bedroom will likely fail or at least be compromised. You will note from the figure that as a complexity becomes less “well,” the relational area becomes progressively larger because there are always more ways for things to go wrong than “more right” just as there were many more ways for a room to be messy than tidy. Also, just as with a room destroyed by a tornado, there are a near infinite number of ways for a complexity to be in a “destroyed state.”
Figure 3.
Each “column” in figure 3 abstractly represents a system that is progressively less ordered, or higher in entropy as one goes from left to right. Consistent with this degree of order, the triangles are progressively less “restricted” in their relationships going from left to right, until it is “destroyed” at right. The equilateral triangle on the left is most ordered, and its relational space is smallest as well – as depicted by the small area of its relational “square.” There are a greater number of possible relata for any triangle where the ends meet as in the 2nd column from the left, and ever greater numbers of relata and larger relational spaces as the lines simply have to cross each other, and finally are fully disassociated. Similarly, for a “tidy” room or a “well” system like a healthy human, there are fewer ways for its constituents to be in relationship to each other, whereas there is essentially an infinite area of a system that has been destroyed. Note that the relational spaces are not drawn to scale but should be successively much, much larger.
The main point here is that complex systems must similarly have its components ordered in a relatively small (but not singular) number of ways to function well. The farther that set of relationships varies from that ideal set of relationships, the more likely it is to not function well, and eventually fail to even be a recognizable system.
The main point about understanding complex systems, at least on a fundamental level, is that the problems that philosophy seeks to address usually occurs in the context of complex systems. Failing to take that into account will nearly always lead to only partial solutions. Admittedly, it is not easy to place philosophical queries and theories into the context of complexities, but doing so is worth the effort as I hope my little philosophical forays will demonstrate. I also recommend reading books on complexity or attending a course offered by the Santa Fe Institute. I would even suggest that having a good understanding of complex systems should be one of the core subjects of any liberal arts education. After all, the world is fundamentally complex and ignoring that fact leads to an incomplete understanding of how the world functions, and importantly, contributes to countless blunders.
References:
1. (Mitchell, M (2009), “Complexity – A Guided Tour,” Oxford, UK, Oxford University Press’ Waldrop, MM (1992), “Complexity – The Emerging Science at the Edge of Order and Chaos,” New York, Simon & Schuster).
2. “What is a Complex System:” 1. “Complexity – A Guided Tour” by Melanie Mitchell, 2. “Simply Complexity” by Neil Johnson, 3. “Understanding Complexity” by Scott E. Page, article, Ladyman, J., Lambert, J., Wiesner, K., and, 4. “What is a Complex System?, PhilSci Archive, 2011, accessed 9/14/2020, http://philsci-archive.pitt.edu/id/eprint/9044.
3.What is Life? Erwin Schrodinger, Cambridge University Press. p69, 1944.
4. Berwald, J, “The Immortal Jellyfish, Discover Magazine, 11/9/2017, see: https://www.discovermagazine.com/planet-earth/the-immortal-jellyfish , accessed 12/1/2020.
5. Gleick J (2008), “Chaos – Making a New Science,” New York, Penguin Books, p20-21

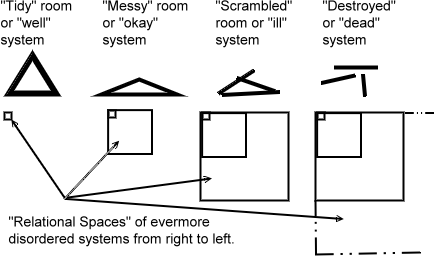